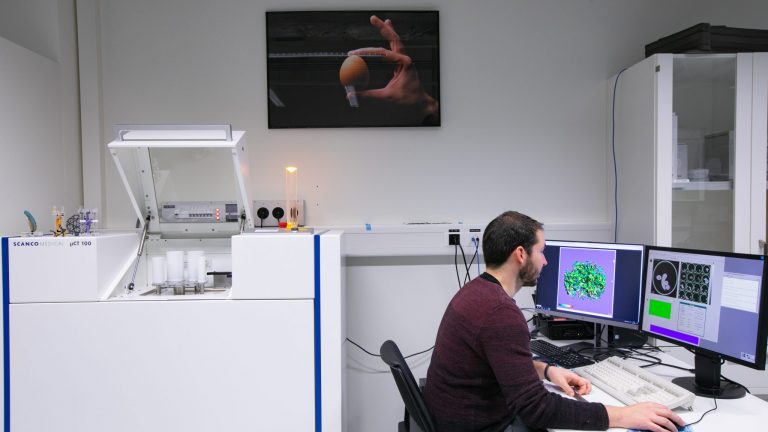
PhD in the Flexible Structures Laboratory (fleXLab)
Mechanics of Physical Knots: From Filaments in Contact to Surgical Suturing
In this thesis, the mechanics of tight physical knots was studied. Knots are omnipresent in surgery, climbing, and sailing, with disastrous consequences when the filament or the rope fails to perform its function. Existing models in knot theory or Kirchhoff’s theory for elastic rods are often insufficient to describe the functional behavior of tight physical knots due to the intricate three-dimensional nonlinear geometries, large elastic (and sometimes plastic) deformations, and frictional interactions.
To address these challenges, we employed a comprehensive approach combining topological and geometric arguments, precision-model experiments, and high-fidelity numerical simulations. We developed an elaborate toolbox for volumetric imaging of X-ray micro-computed tomography data, enabling detailed analysis.
Firstly, we conducted a compare-and-contrast examination of the equilibrium shapes of physical and ideal trefoil knots in various configurations. Second, we investigated the performance of stopper knots, which prevent the rod end from retracing through a narrow passage. Third, we studied the operational and safety limits of surgical sliding knots, highlighting the previously overlooked but crucial effect of plastic deformation. Finally, we found that all the experimental and numerical data, involving all the knot configurations we investigated and a wide range of friction coefficients, collapse onto a master curve.
The acquisition and study of unprecedented experimental data, combined with FEM simulations, has enabled us to systematically explore the different ingredients dictating the mechanical performance of knotted structures with highly nonlinear geometrical features and material properties.
Paul is now working as an R&D Engineer for mechanical watch movements at ETA SA – Manufacture Horlogère Suisse. When not tackling large-scale chronometric challenges in the watchmaking assembly lines, he investigates the intricacies of isochronism, pushing the boundaries of traditional chronometric theory.
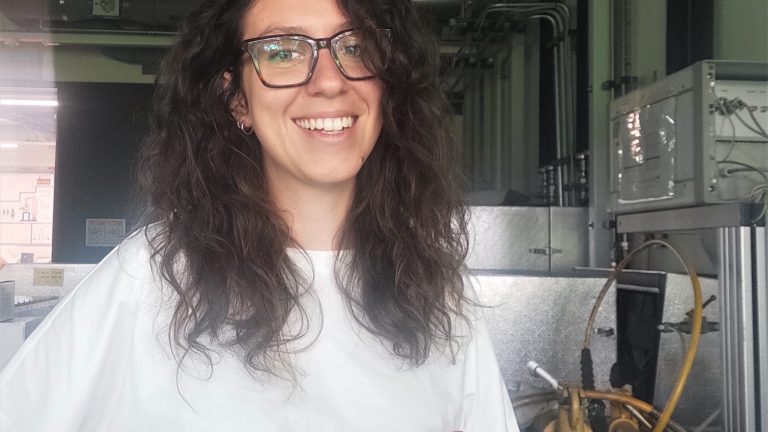
PhD in the Laboratory of Experimental Rock Mechanics (LEMR)
Nucleation, propagation, and scale dependence of laboratory frictional ruptures; implications for earthquake mechanics.
Earthquakes, as natural phenomena, pose significant challenges due to their complex mechanics. Despite considerable progress in understanding their initiation and propagation, many aspects remain insufficiently detailed. Seismic ruptures, resembling both propagating cracks and sliding processes governed by friction, present an intriguing duality. My thesis employs experimental methods to deepen our understanding of this dual nature and explore its implications, particularly regarding scale dependence and the emergence of rupture complexities.
We initially explore how off-fault measurements can detect precursory phases of earthquakes by monitoring seismic properties over time. Next, we investigate the impact of lubricants, akin to natural and industrial fluids in faults, which promote fault reactivation and alter rupture propagation characteristics. Furthermore, we delve into the difference between fracture energy and breakdown work, highlighting the role of frictional weakening and its effect on rupture dynamics. Our findings reveal unconventional singularities arising from frictional weakening, such as flash heating, which significantly influence rupture behavior. Lastly, using a newly developed large biaxial apparatus, we examine the scale effects of frictional ruptures on fault systems larger than the characteristic nucleation size, uncovering complexities in their reproduction at larger scales.
After completing the Ph.D., I was granted the SNSF (Swiss National Science Foundation) Early Postdoc Mobility Fellowship to work in the Earthquakes team of Géoazur, Université de Côte d’Azur, France, as a postdoctoral researcher. My current research work focuses on the dynamics of elongated ruptures.
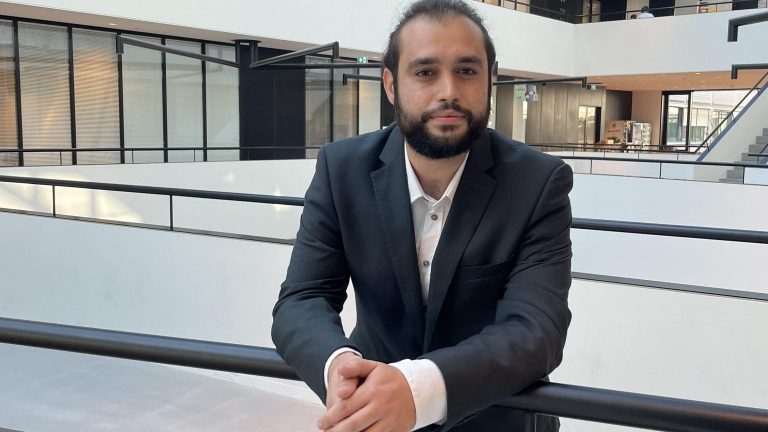
PhD in the Laboratory of Fluid Mechanics and Instabilities (LFMI)
From coating flow patterns to porous body wake dynamics via multiscale models
Multiscale phenomena are involved in countless problems in fluid mechanics. Their intrinsic complexity given by the interplay of two or more length scales is illustrated by the intricate patterns observed in coating flows, e.g. wine tears in a glass and dripping of fresh paint applied on a wall, and by the stable flight of dandelions seeds, transported by the wind thanks to a hairy, permeable, structure, so-called pappus.
The thesis investigates the pattern formation of several coating flows and the wake dynamics past diverse permeable bodies via multiscale models. We show that the flow of a thin below an inclined substrate is dominated by the presence of free-surface structures modulated along the direction transversal to the main flow, called rivulets, which may destabilize with the formation of traveling drops. We determine the critical values of the inclination angle and film thickness beyond which rivulets destabilize and drip. These elongated, streamwise-aligned, structures grow also on the substrate when a deposition law mimicking the growth of karst structures is considered.
The role of modifications of the substrate is then investigated in the cases of dewetting of very thin polymer films, in the context of
production of optical metasurfaces, and in the case of three-dimensional spreading of a mass of fluid, reminiscent of environmental problems.
The last part of the thesis is devoted to the modifications of wake flow instabilities past bluff bodies when composed of a permeable microstructure. We also show that permeability ensures a stable flight for very permeable disks.
Despite the broad range of problems considered, we show that multiscale models can capture the intricate patterns of these flows.
Pier Guiseppe is now working in the Department of Environmental Civil Engineering and Architecture (DICAAR) at the University of Cagliari, Italy. His research mainly focuses on cardiovascular and environmental fluid mechanics.
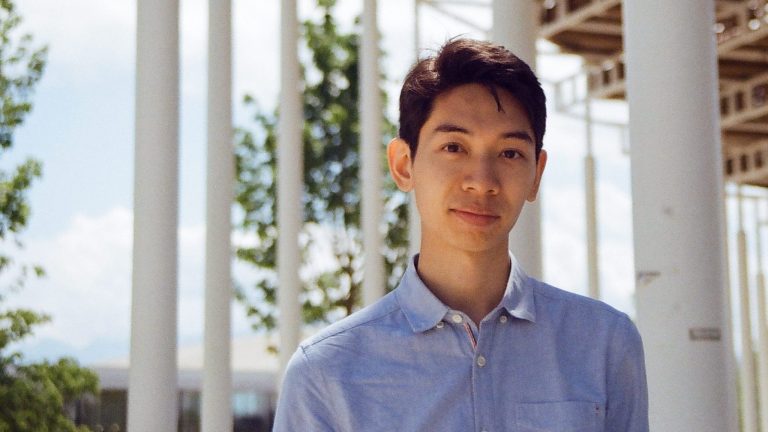
PhD at the Computational Solid Mechanics Laboratory (LSMS)
Modeling the origins of adhesive wear across scales
Friction and wear occur at every interface between solid materials, for example in mechanical devices with moving parts, impacting their function and lifetime. They also appear at a much larger scale in geological faults, contributing to the complexity of seismic phenomena. Simple models exist to describe friction and wear, like Coulomb’s friction law and Archard’s wear model. However, such models are empirical, meaning they must be tuned with experimental measurements, that are not always available. From a scientific point of view, these models give little to no insight into the mechanisms involved. My thesis aims to enhance the current understanding of dry friction and wear at a more fundamental level, regardless of the materials and geometries involved, starting with considerations at the atomic scale and building a bridge toward larger scales.
Using numerical tools and experiments, we found a microscopic explanation for the origin of wear, which starts with the formation of fine particles whose size depends on the details of the interface’s geometrical configuration. The wear particles then gradually agglomerate to create a macroscopic surface roughness. By numerical means, we also established a direct link between surface roughness and wear volume achievable inside a contact, giving a mechanistic explanation for the emergence of the severe wear regime, experimentally observable. A novel numerical method based on discrete elements was developed to tackle microscale wear modeling much more effectively than with molecular dynamics, a numerical method commonly used for this problem but bearing a significant computational cost. Finally, using some of the knowledge earned along the way, a practical case was investigated, involving oxidized silicon, a material typically used in electronics but findings its way toward mechanical applications.
I am now part of the ENAC-IT4R group at EPFL as a software engineer, providing services to the research laboratories within the department of architecture, civil, and environmental engineering.
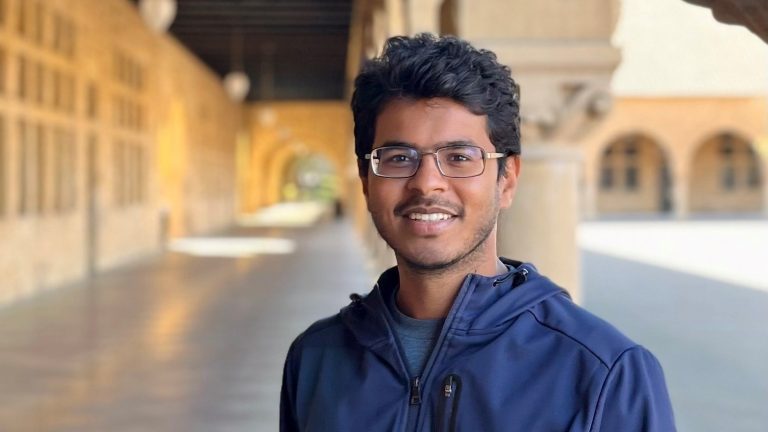
PhD in the Laboratory for Multiscale Mechanics Modeling (LAMMM)
“Atomic Scale Investigations into the Origins of Ductility in Mg Alloys”
When we hear the word crystal, an array of atoms arranged on a neat periodic grid immediately comes to mind, exemplified by Al, Cu, Mg, Fe, etc. Several important properties of crystals that make them ubiquitous in our daily life derive from imperfections and defects present in crystals. Understanding, controlling, and engineering these crystalline defects is crucial for developing, optimizing, and designing novel classes of technologically important materials.
My Ph.D. research focuses on the behavior of linear defects called dislocations – principal carrier of plastic deformation in crystals- in Mg and its alloys. Mg is a lightweight, biocompatible, and abundantly available crystalline material that holds a vast promise for manufacturing energy-efficient transportation. However, its intrinsically low ductility and low fracture toughness severely hamper its large-scale industrial application as a structural material. The behavior of Mg dislocations is at the heart of this problem. The underlying hexagonal close-packed (HCP) crystalline structure of Mg renders specific types of dislocation, pyramidal <c+a> dislocation, immobile that, in turn, results in low ductility of pure Mg. The addition of some elements, including Ce, Ca, Y, Nb, Zr, etc, enhances the ductility of resulting Mg alloys by activating the pyramidal <c+a> dislocations. The Ph.D. thesis proposes a mechanism by which various alloying elements accelerate the cross-slip process – a mechanism by which a dislocation changes its glide plane – to increase the activity of the pyramidal <c+a> dislocations. Various aspects of the proposed mechanism are investigated by conducting atomistic simulations. Finally, a mathematical model is proposed that helps us screen the alloying elements and their concentration to design and optimize new Mg alloys with desired properties.
After completing the Ph.D., I was granted the SNSF (Swiss National Science Foundation) Early Postdoc Mobility Fellowship to work in the Micro and Nano Mechanics Group at Stanford University as a postdoctoral researcher. My current research work focuses on utilizing machine learning techniques to investigate the thermodynamic properties of crystalline materials.
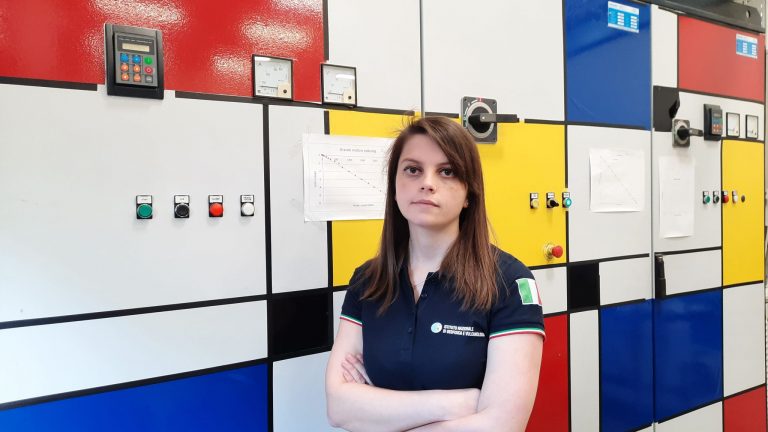
PhD in the the Laboratory of Experimental Rock Mechanics (LEMR)
“Mechanical behaviour of fluid lubricated faults during earthquake nucleation and propagation”
One of the most alarming recent findings in geoscience is the worldwide increase in human-induced seismicity. The latter is due to engineering operations in deep reservoirs for hydrocarbon production, wastewater and CO2 storage and exploitation of geothermal resources which result in the reactivation of nearby faults. The seismic reactivation of faults due to fluid pressure has been extensively studied, but the influence of the injected fluid has been overlook. For example, the viscosity of injected fluids can vary of several orders of magnitude. In my PhD thesis, supervised by Prof. Marie Violay, we aim at understanding the influence of fluids viscosity on the phases of the seismic cycle, in particular during seismic slip nucleation, reactivation and propagation using laboratory experiments and numerical models.
Currently, I am working at the Istituto Nazionale di Geofisica e Vulcanologia (INGV) in Rome as a postdoctoral researcher. I am manly focused on the study of upscaling seismic laboratory results to natural scale using physics-based numerical models. I am also involved on the ERC Synergy project FEAR, which aims to investigate induced seismicity and earthquake physics at the meso-scale.
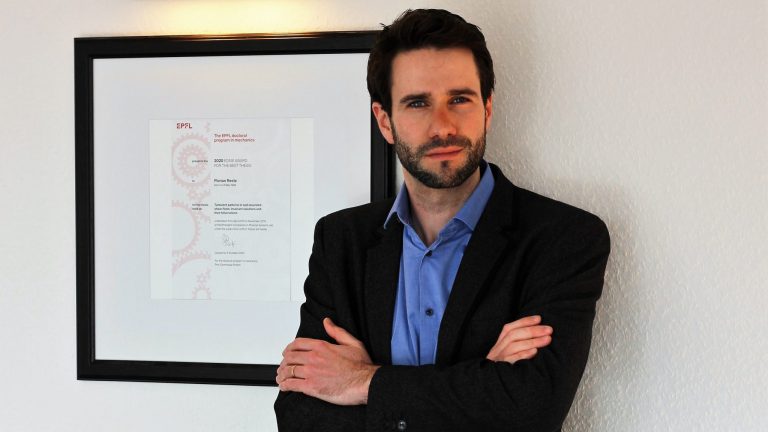
Ph.D in the Emergent Complexity in Physical Systems Laboratory (ECPS)
“Turbulent patterns in wall-bounded shear flows: invariant solutions and their bifurcations”
When wall-bounded shear flows become turbulent, the flow may self-organize into characteristic spatially periodic patterns of unknown origin. To understand how regular patterns emerge in a turbulent flow, a nonlinear theory is needed. In my Ph.D thesis, we construct and analyze exact invariant solutions of the 3D nonlinear fluid flow equations that capture the non-trivial spatial structure of patterns in turbulent flow. This approach requires high-performance computational tools, that have been developed as part of my thesis and are now publicly available within a widely used state-of-the-art open source software.
Using the developed numerical methods, turbulent patterns in two types of wall-bounded shear flows have been studied: oblique turbulent-laminar stripes in plane Couette flow and a multitude of convection patterns arising in inclined layer convection when the angle of inclination is varied. The existence and properties of exact invariant solutions underlying these patterns leads to a better understanding of the complex spatio-temporal dynamics in spatially extended turbulent flows.
Now I am working as a software engineer at PSI Software AG in Berlin on forecasting renewable energies and their influence on our electrical grid.
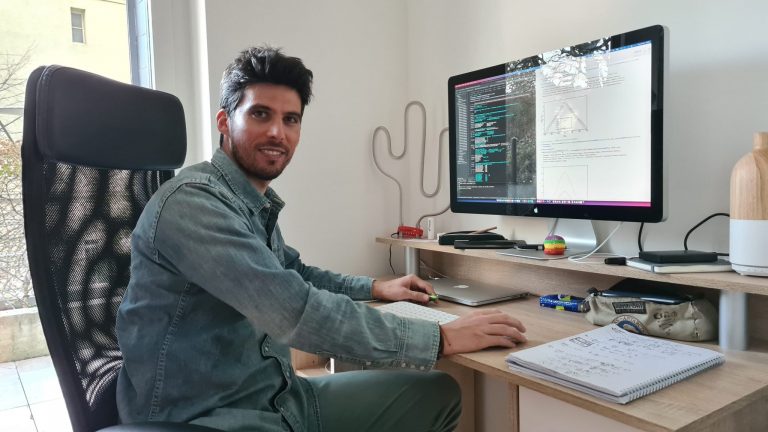
PhD in the Geo-Energy Laboratory (GEL)
“Hydraulic stimulation of pre-existing discontinuities in tight rocks”
Deep geothermal energy represents a promising source of renewable energy that might play a key role in the World’s future energy balance. It is a constant resource, largely available across continents that can be used to supply heat and possibly electricity. Despite its great potential, there are still some issues that prevent its diffusion and employment. Perhaps, one of the most important is induced seismicity that tremendously affects public safety as well as its opinion on this type of green energy. In my PhD thesis supervised by Prof. B. Lecampion, we proposed theoretical models and specific numerical algorithms that can help understanding the role of fluid injection and propagation at depth on the transition between a-seismic and seismic deformations.
Currently, I am working at Swiss Seismological Service (SED) at ETH Zürich as postdoc associate. My research activity is mainly about numerical modelling of induced seismicity using empirical, hybrid and physic-based models.
I am also involved in an international project called “Innovation for De-Risking Enhanced Geothermal Energy Projects (DEEP)” where I am responsible for the development of new generation of induced seismicity forecasting models based on Deep Learning techniques.
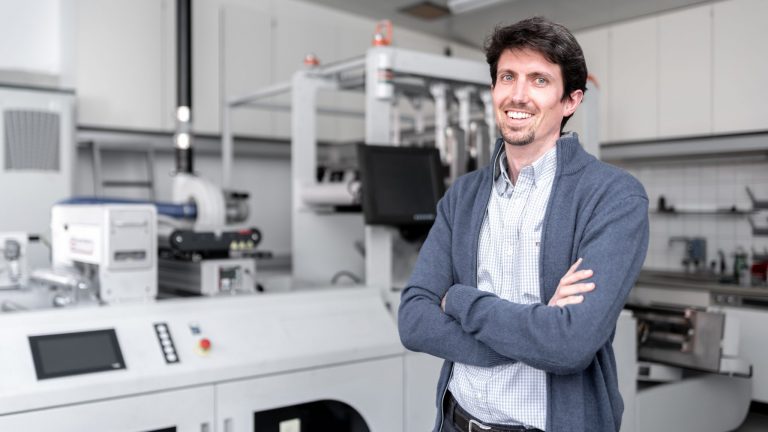
PhD in the Laboratory of Fluid Mechanics and Instabilities (LFMI)
“Pattern formation in thin liquid films: from coating-flow instabilities to microfluidic droplets”
Nature and industrial applications abound with thin viscous flows, ranging from the lava flow on volcanoes to the lubricating layer around confined droplets in microchannels. We all have already observed that thin-film flows often form well-defined patterns, as the tears in a glass of wine, or the crystalline-like pattern of pendent droplets under the kitchen lid.
The aim of this thesis is to investigate the mechanisms underpinning the pattern formation in thin-film flows for different configurations. A theoretical model is developed and complemented with advanced stability analyses, numerical simulations and experiments.
We show that the gravity-driven instability of a liquid film under curved or inclined substrates is at the origin of the fascinating karst formations encountered in limestone caves. Furthermore, we find and rationalize a stable regime of these film flows that can be harnessed to produce hemispherical elastic shells in a very robust and versatile manner.
We also provide a detailed characterization of the thin-film profiles around confined droplets, key for a better prediction of their dynamics in microfluidic devices.
In spite of the broad range of scales considered, we show that all the treated problems and the pattern selections can be described by similar lubrication equations.
Gioele is now co-responsible of the iPrint Institute and Assistant Professor at the HES-SO University of Applied Sciences and Arts of Western Switzerland in Fribourg (HEIA-FR), where he teaches fluid mechanics classes. His research is focused on the development of new digital printing and manufacturing techniques and processes.
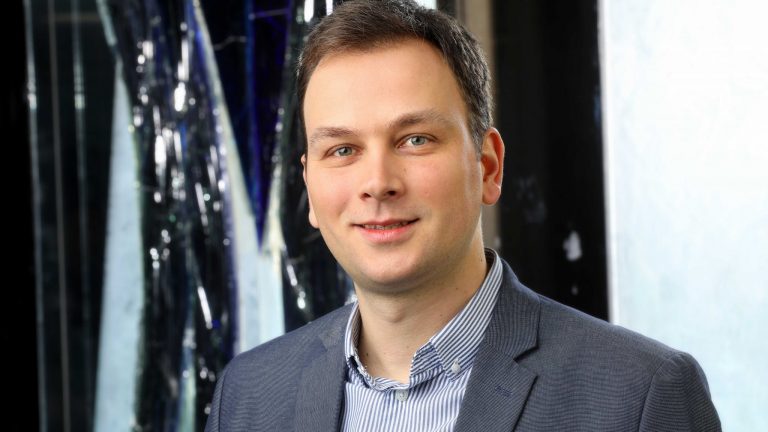
PhD in the Laboratory for Multiscale Mechanics Modeling (LAMMM)
The mechanics of crack-tip dislocation emission and twinning
This thesis proposes two new theories for describing the mechanics of crack tip dislocation emission and twinning. Dislocation emission from a crack tip is a necessary mechanism for crack tip blunting and toughening, and at the same time one of the classical problems in mechanics of materials. A material is intrinsically ductile under Mode I loading when the critical stress intensity factor for dislocation emission KIe is lower than the critical stress intensity factor KIC for cleavage. KIe is usually evaluated using the approximate Rice theory. Atomistic simulations have shown that this theory is reasonable but not highly accurate. The discrepancy arises because Mode I emission is accompanied by the formation of a surface step that is not considered in the Rice theory. In this work we propose a new theory for Mode I emission based on the idea that (i) the stress resisting step formation at the crack tip creates “lattice trapping” against dislocation emission such that (ii) emission is due to a mechanical instability at the crack tip. Furthermore, a theory for crack-tip twinning has been proposed accounting for (i) the absence of the step formation, and (ii) the fact that nucleation does not occur directly at the tip. Both theories are quantitatively validated against atomistic simulations across a wide set of fcc materials.
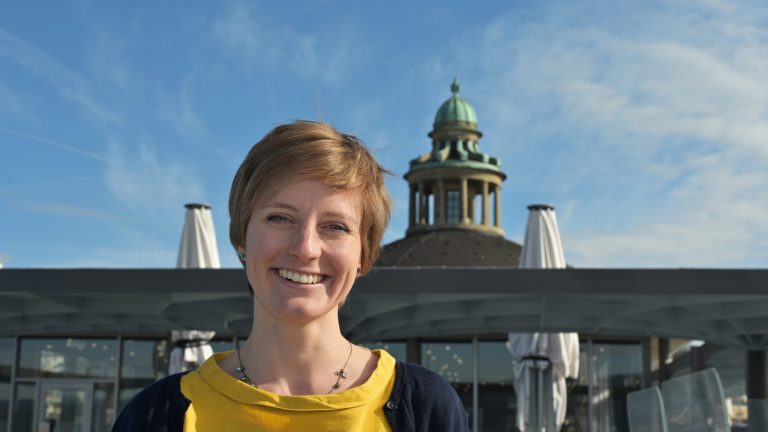
Phd in the Laboratory for Hydraulic Machines (LMH)
“Collapse phenomena of deformed cavitation bubbles”
After completing my PhD in the laboratory for hydraulic machines (LMH) under the supervision of Mohamed Farhat, I obtained an SNSF Early Postdoc Mobility fellowship to work at the University of Colorado Boulder, USA. This experience allowed me to apply the knowledge that I had gained during my PhD on the fluid dynamics of cavitation bubbles to studies of ultrasound contrast agent microbubbles in biomedical applications.
I have since returned to Switzerland as an assistant professor of multiphase fluid dynamics at ETH Zurich, where I am building a lab to continue with experimental research activities involving fast fluid dynamics at small scales.