Gas-Water interface Dynamics – Crossing a Molecular Beam and a Liquid water jet
We are interested in chemical reactions at liquid water surfaces. Despite their importance on earth and in our atmosphere, such processes are poorly understood, primarily because until recently no experimental technique was available that would enable the combination of molecular beams, required for a controlled preparation of the gas-phase molecules, with liquid water surfaces.
We are combining traditional molecular beams with so-called liquid-microjets to obtain a clean reaction environment that sustains both the gas and the liquid phase in the desired conditions. Reaction products are detected as a function of time, mass, and scattering angle in order to reconstruct a complete image of the dynamics at the surface. In this manner we can distinguish between elastic scattering, absorbtion/desorption processes, and chemical reactions happening at the surface.
To perform this research we have initiated a D-A-CH network, funded by the DPG and the SNF, wherein we collaborate with Dr. Bernd Winter (Fritz-Haber-Institute of the Max-Planck Society, Berlin) and Prof. Bernd Abel (University of Leipzig)
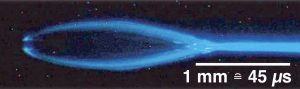
Kinetics at liquid-liquid interfaces
We use liquid flat jets to prepare a controlled interface between two miscible liquids. If different reactive solutes are contained in the two solutions from the two cylindrical jets, then diffusion across the interface can lead to reaction. Because the flow speed of the solutions is constant, a particular point along the jet axis always corresponds to a specific time after the liquid has left the jet, thus creating a direct relation between position in the jet, and time of interaction. This in turn allows us to relate observed reactant and product concentrations to time which gives direct access to the reaction kinetics.
We demonstrate this approach using the well-known Luminol oxidation reactoin which yields an electronically excited that radiatively decays to the ground state. This chemiluminescence is used as a marker for the product concentration and serves to monitor the kinetics of the reaction (JACS 144, 7790 (2022))

Cold and Controlled Chemistry – Merged Beams
Our main interest are the reaction dynamics of neutral molecules at temperatures around and below 1 K. At these temperatures, the collision properties between the reaction partners can be dominated by quantum mechanical effects that are not observable at higher temperatures.
The good theoretical understanding of these processes stands in sharp contrast to the experimental data where almost nothing is available. The main reason for that is the difficulty in the preparation of neutral molecules at translational temperatures below 1 K.
Different methods have been proposed and implemented to obtain molecules in the desired temperature range. In our group, we pursue different tracks to obtain cold neutral molecules and use them in low-energy collision studies.
Gas phase collision experiments traditionally are performed in crossed molecular beams. This technique has been developed several decades ago and provided some of the most fundamental insight in reactive scattering dynamics. For the particular application to low temperatures, or low collision energies, however, crossing two beams proves difficult.
In order to reduce the collision energy, the relative velocity has to be reduced. For a crossed-beam configuration this would require decelerated molecular beams, and the deceleration would need to be maximum to achieve the lowest temperatures. Zero relative velocity is not feasible since the molecules always need to move in order to reach the interaction point.
Our solution to circumvent this problem is to transfer the collision from the laboratory frame of reference into the molecular frame by performing merged-beams experiments instead of crossed beams. By merging two beams, i.e., overlaying them in a manner that they also move along with each other, the only parameter relevant for the collision energy is the relative velocity. The beams can still move at high velocity in the laboratory.
We have implemented this technique by using two bent guides, one electric and one magnetic, to steer and merge two supersonic beams, one polar and one paramagnetic. By adjusting the conditions of the expansions, the relative velocity is controlled and the collision energy can be set anywhere between several tens of K and zero (J.Chem.Phys.; EPJTI).
Using specially designed electro magnets we can furthermore orient the paramagnetic particles emerging from the magnetic guide. In this manner we can study the stereochemistry of reactions between paramagnetic particles and other atoms or molecules in the same energy range, and in particular at collision energies below 0.1 K (Phys. Rev. Lett. 2017; J.Chem.Phys; Nat. Chemistry, Phys. Rev. Lett. 2019).
3D printing of electrostatic guides
We have recently demonstrated a new approach to the production of conductive structures for our experiment: the 3D printing of a plastic piece, followed by selective electroplating (see our paper in Phys. Rev. Applied, highlighted on the APS Physics blog). Using this approach we have build a beam splitter for polar molecules. This device, shown in the photo above, initially confines molecules transversely in an electrostatic hexapole guide that then smoothly converts into two bent quadrupoles. The initial molecular beam is evenly divided among the two arms, and two separate bags result that are correlated in terms of particle density and temperature.
3D printing offers several important advantages over traditional machining, for example dramatically reduced production cost and time, but also a much larger flexibility in terms of shapes that can be fabricated.The particular structure required for the beam splitter includes several curved and split electrodes that would be very difficult to make by traditional means. Furthermore, the electroplating provides inherently smooth surfaces that require no further polishing and guarantee the compatibility with very strong electric fields, as required for the guiding of polar neutral molecules.
The combination of 3D printed plastic pieces with electroplating opens many new possibilities, not just in gas-phase molecular physics but in any other field where structures with high geometric complexity, electrical conductivity, high-quality metal surfaces, high precision, and vacuum compatibility are required.
Details about and results from our experiments can be found in the list of recent publications.