Research
Our research is dedicated to solve a wide range of problems in biological and living systems and soft and active materials, spanning from inert materials of foam, emulsions, polymeric gels, and granular materials to active living materials of bacteria aggregates and biological tissues. Our primary goal is to gain a deeper understanding of the fundamental principles governing the observed phenomena within these systems by developing novel theoretical framework based on experimentally observed features.
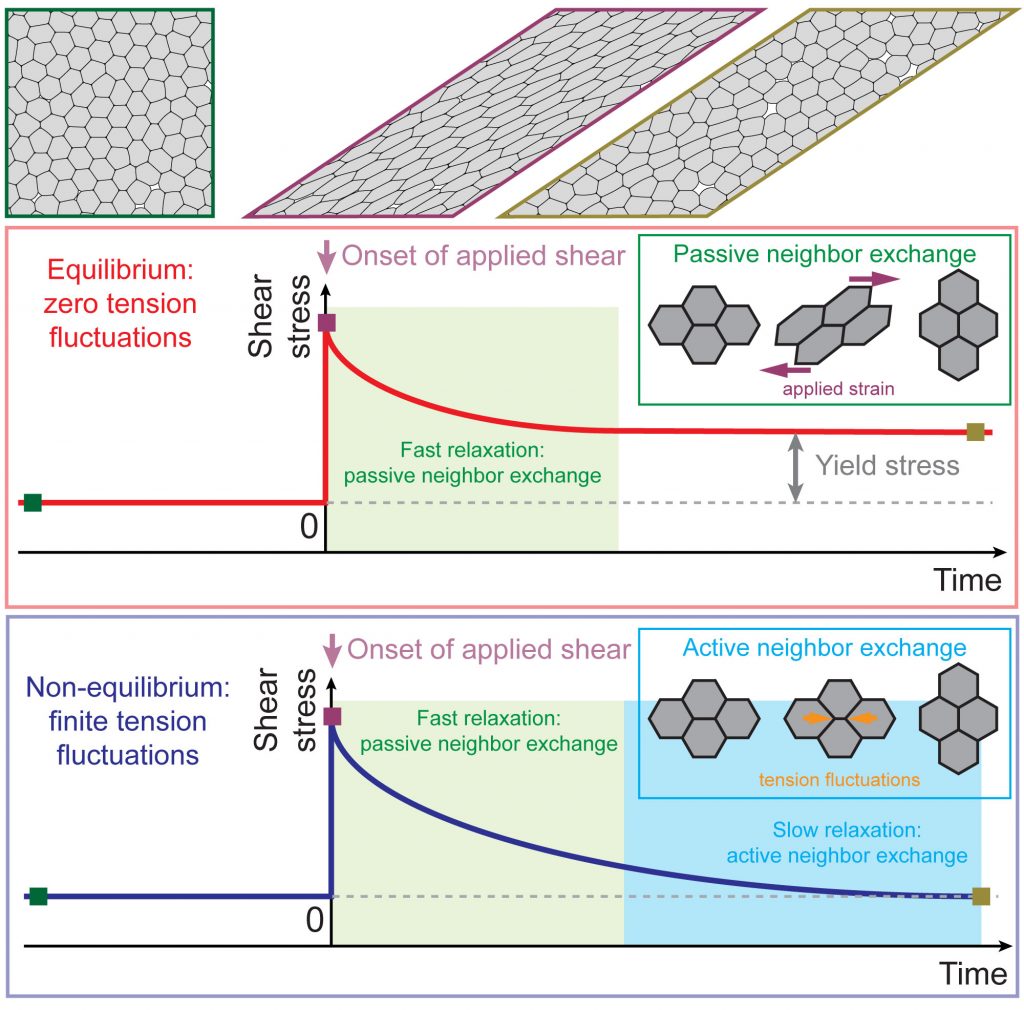
Rigidity transitions in embryonic tissues. Shear simulation configurations: no applied strain (top, left), onset of applied strain (top center), and relaxed state after applied strain (top right). Stress relaxation and tissue fluidization behaviors in equilibrium systems(middle) and non-equilibrium systems (bottom), showing that solid-like states are characterized by finite yield stress in equilibrium systems while the comparison of time scales is necessary to distinguish solid/fluid states in non-equilibrium systems as the residual stress is all relaxed by active fluctuations.
S. Kim, M. Pochitaloff, G.A. Stooke-Vaughan, and O. Campàs, “Embryonic Tissues as Active Foams”, Nature Physics, 2021, 17, 859-866.
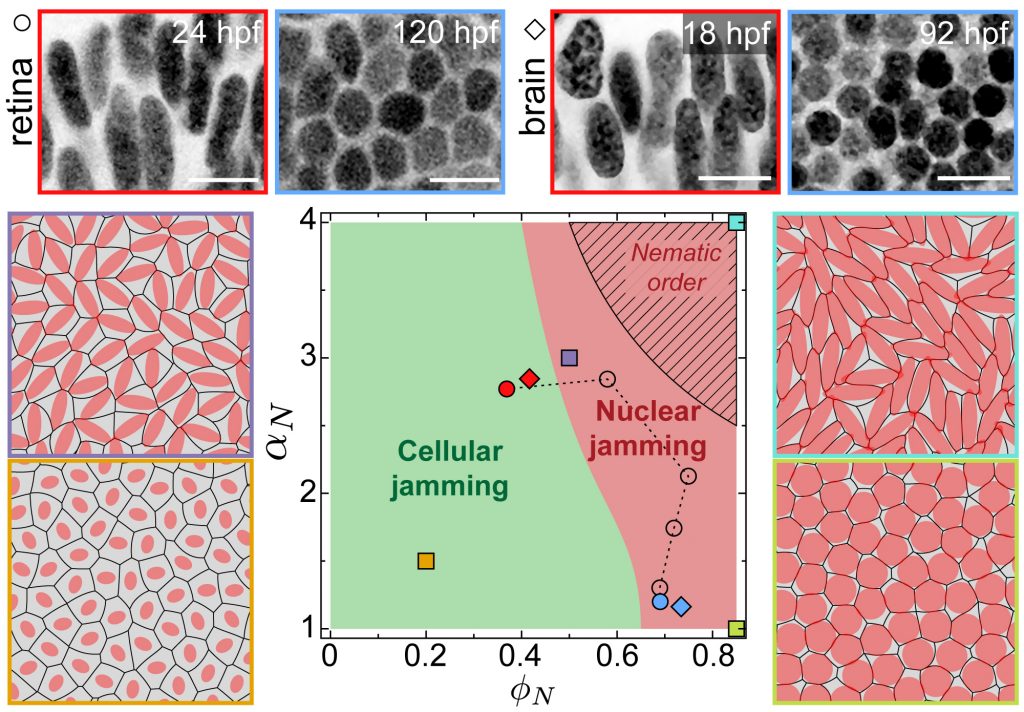
We show that (1) for round nuclei, cell movement progressively slows down and tissue structure becomes more ordered as the nuclear volume fraction increases over the critical value, (2) the critical volume fraction for the dynamic slowdown decreases for an increasing nuclear aspect ratio, and (3) the formation of local nematic domains at a large nuclear volume fraction and a large nuclear aspect ratio enhances cell movement along the major axis direction of nuclei. Structural analysis of eye and brain tissues in zebrafish shows that these tissues undergo a nuclear jamming transition. Our result reveals a novel nuclear jamming transition, highlighting the importance of nuclei in the control of emergent tissue mechanics and tissue structures.
S. Kim, R. Amini, and O. Campàs, “A nuclear jamming transition in vertebrate organogenesis”, BioRxiv, 2022
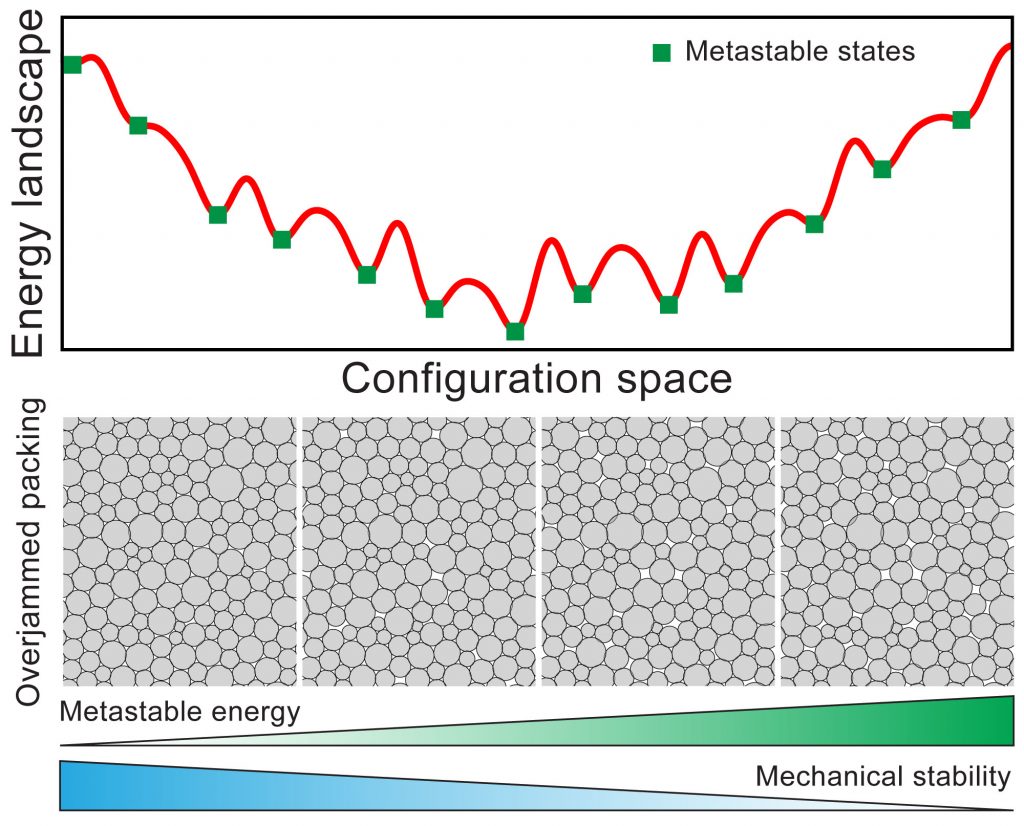
Numerous soft condensed matter systems are characterized by a complex energy landscape with a huge number of metastable states. Due to the complexity of the energy landscape, it is extremely difficulty to approach the ground state, so many experimental systems commonly correspond to one of the metastable states in mechanical equilibrium. As physical/mechanical properties of these materials strongly depend on the energy level as well as structural properties, it is important to understand how the energy level, the structural features, and emergent material properties are all connected to each other for different metastable states.
In a large class of soft-disk packings, we find that (1) structural measures, which can be evaluated from a snapshot of metastable states, can predict the energy level of metastable states. (2) low energy metastable states exhibit superior mechanical stability under shear deformation, and (3) these low energy metastable states can be efficiently constructed, using structural measures as a guide to the optimal reference packing structure. This work can be utilized as design principles to construct smart materials and metamaterials of desired mechanical properties and also can be applied to understand low temperature equilibrium states in glasses and supercooled liquid.
S. Kim & S. Hilgenfeldt, “Structural Measures as Guides to Ultrastable States in Overjammed Packings”, Physical Review Letters, 2022, 129, 168001
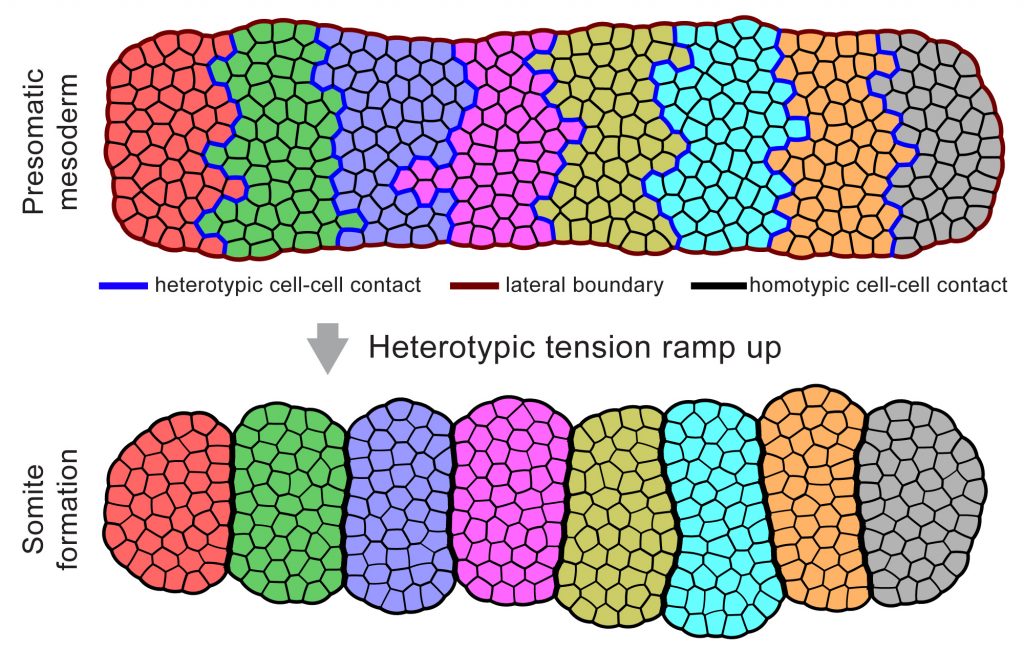
The formation of somites is a key process in vertebrate development. During somitogenesis in zebrafish embryo, the presomitic mesoderm transforms into a periodic, bilaterally symmetric pairs of somites that divide the anteroposterior (heat-to-tail) axis. While synchronization of genetic oscillators is known to play an important role in segmentation, the physical mechanisms underlying somite formation are unknown.
We investigated the physical aspects of somite formation by combining in-vivo experiments and numerical simulations of the mechanical model. We experimentally observe an accumulation of myosin and F-actin at the forming somite boundary, which indicates an increase of effective boundary tension. Extending the Active Foam Description to account for the geometry of the tissue, we find that (1) the right amount of tension increase at the somite boundary is necessary to induce the observed boundary shortening and straightening by plastic cell rearrangements, and (2) an optimal level of junctional tension fluctuations is required to maximize robustness of somitogenesis. The combination of experimental data and numerical simulations elucidated a key physical mechanism of the somite formation and provided a solid example of applicability of the Active Foam Description.
E.R. Shelton, S. Kim, B.J. Gross, R. Wu, M. Pochitaloff, I. Lim, E.M. Sletten, O. Campàs, “Stress-driven tissue fluidization physically segments vertebrate somites”, BioRxiv, 2021