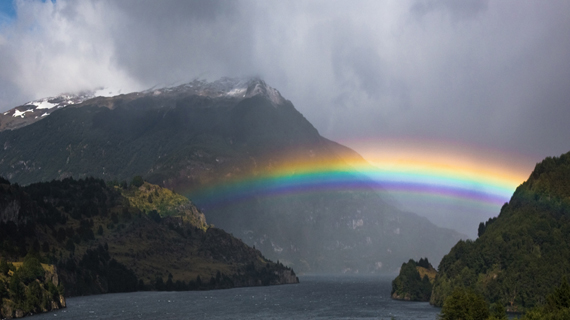
Our perception of the world is based on linear optics, where different optical waves do not interact and behave independently: in a rainbow, you can consider the path taken by one color individually, or by another color individually, or consider all the colors together, you will always get the same result.
This is not the case when you work with very short (a few 100s fs) and very intense pulses of light. In this case, some amazing nonlinear effects can occur, like the generation of second harmonics: two low energy photons (= long wavelength, say 800 nm) can add up to produce one high energy photon with half the wavelength (400 nm). In other words, light in the deep red can converted into violet light.
Classically, this phenomenon requires some special, dielectric, nonlinear crystals. At the NAM, we explore the possibility of using plasmonic nanostructures to produce such nonlinear effects.
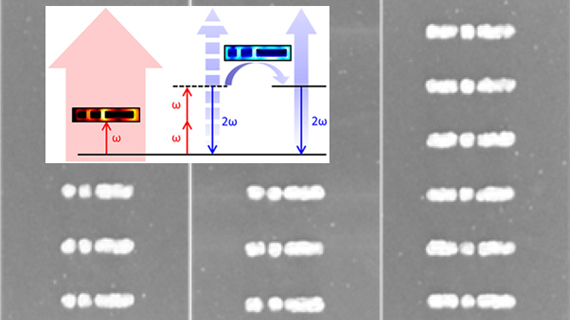
Since second harmonic generation requires photons at both the fundamental frequency ω and the second harmonic 2ω, we have devised aluminum optical antennas that have two separate resonances at these two different frequencies. The previous image shows such a sample and explains the enhancement mechanisms for second harmonic generation. When the geometry is tuned such that both resonances are strong, the second harmonic is very significantly enhanced. Note that a specific technology had to be developed to produce good nanostructures in aluminum, a very good metal for nonlinear optics, but quite difficult to work with.
In nonlinear optics. the momenta of the different waves involved play a key role. This is illustrated in the following experiment conducted in collaboration with Mikael Käll at Chalmers University: the optical second harmonic generation from plasmonic metasurfaces composed of aligned gold nanopillars with a pronounced out-of-plane tilt demonstrated that these samples function as wavevector-selective nonlinear metasurfaces, that is, the coherent second harmonic signal does not only depend on the polarization and wavelength of the excitation beam, but also on its direction of incidence, in spite of the subwavelength thickness of the active layer. Specifically, the nonlinear response can vary by almost two orders-of-magnitude when the incidence angle is changed from positive to negative values compared to the surface normal.
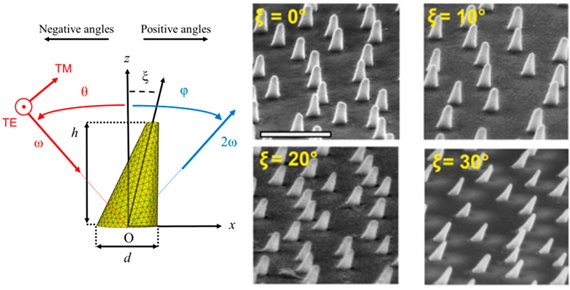
Frequency up-conversion
Second harmonic generation is a coherent process that requires short laser pulses and produces mainly photons at exactly twice the excitation frequency (although a less strong, broadband luminescence spectrum is also often measured). Frequency up-conversion is a non-coherent nonlinear process (like luminescence) that is extremely interesting for harvesting energy from infrared sun light. Indeed, this infrared light has less energy than the gap of most semiconductors used for solar cells and is not converted into current. Using up-conversion, it is possible to transform this low energy radiation into visible light that can then be transformed into current in the solar cell.
At the NAM, we study how plasmonic antennas can enhance the up-conversion process. The figure below shows frequency up-conversion nanoparticles embedded among an array of Ag plasmonic antennas on the left, while the graphs on the right show a very significant increase of light conversion around 550 nm and 650 nm when the system is illuminated in the infrared and the antennas are tuned to the correct resonance conditions.
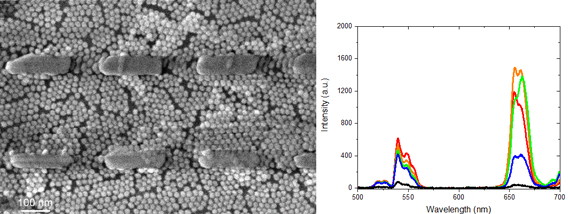