Light is amazing! Not only does it make our days brighter, but its particles have some astonishing properties: the photon does not carry any mass, but yet it has some momentum and it can produce a force on an object. At the NAM, we study different types of forces produced by electromagnetic waves, from the visible range (light) to low frequency microwaves.
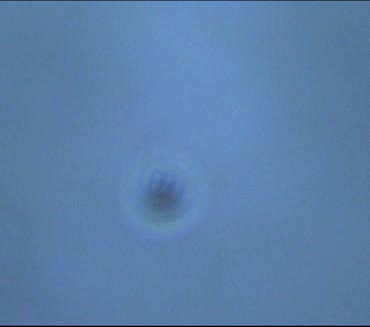
The above movie shows an optical nanomotor that includes a plasmonic rotor embedded into a block of glass. The rotor is shown below on the right: it is about 200 nm wide (you could put 500 such rotors side by side on the section of a human hair!) and has been designed with machine learning to produce an extremely strong torque. As soon as a little light impinges on the nanomotor, it starts rotating. The actual rotation mechanism is also very interesting: the incident light is linearly polarized and does not carry any angular momentum; yet, the scattered light acquires angular momentum and the motor begins rotating by sheer conservation of the momentum (Newton’s third law).
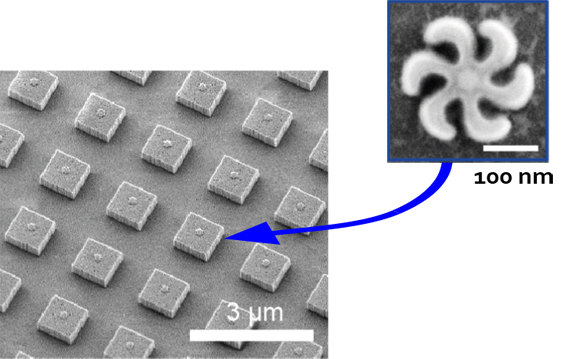
Optical forces can also be used to manipulate objects without touching them physically. So-called optical tweezers are routinely used in biology to move cells around without damaging them. At the NAM, we utilize optical tweezers to probe the chemical environment of a trapped particle, as illustrated below. Here, the motion of a trapped gold nanoparticle is recorded in surfactant solutions with different concentrations. Surfactant molecules – which are just like soap – build some tiny balls called micelles and shown in purple in the image. The motion of the trapped particle depends on the concentration of micelles and by recording its trajectory, we can obtain information on the chemistry of the surrounding medium.
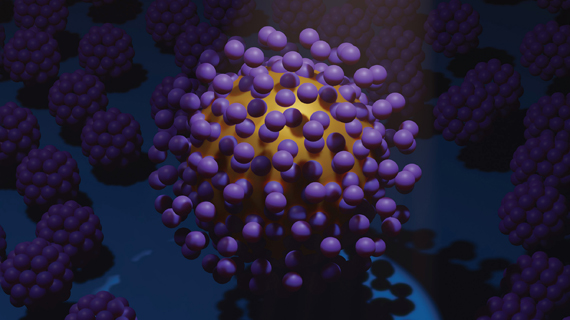
Dielectrophoresis
A very low frequency electromagnetic field (a few kHz) can also produce a force on an object. In this case, one speaks of dielectrophoresis, a topic that we research both theoretically and experimentally. Recently, we have unified the theories of electromagnetic forces over the entire spectrum, from low frequency dielectrophoresis to optical tweezers.
Dielectrophoresis works especially well for manipulating molecular entities like proteins. Since the dielectrophoretic force is proportional to the electric field gradient, we have developed experiments with saw-tooth electrodes of varying spacing that produce different field gradients (the larger the spacing, the smaller the dielectrophoretic force). Such electrodes, illustrated below, can be used to trap a broad diversity of proteins, such as lysozyme, bovine serum albumin, or lactoferrin. Depending on its polarizability, a given protein will be trapped up to a specific spacing, when the dielectrophoretic force becomes too weak to overcome the Brownian motion.
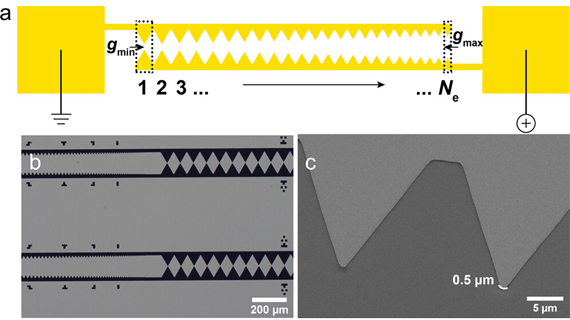